This column is moderated by Béla Lipták, automation and safety consultant and editor of the Instrument and Automation Engineers' Handbook (IAEH). If you have an automation-related question for this column, write to [email protected].
Q: How are they measuring carbon dioxide in the atmosphere? I don’t think any method is accurate enough.
Name withheld
A: Your question is an important one, so I’ll answer it in some detail. CO2 (and other gases) consisting of two or more dissimilar atoms absorb infrared (IR) radiation in a characteristic, unique manner. Water vapor, methane, CO2 and CO are all gases that can be measured with IR sensors. Therefore, IR detectors are is the most widely used for CO2 analyzers. They are stable and highly selective for CO2, they have a long lifetime and, because the measured gas doesn't contact the sensor, they can withstand high humidity, dust, dirt and other harsh conditions.
Precise knowledge of CO2 concentration in the atmosphere is necessary because it has a key role in global warming (Figure 1). Instruments that are used to measure atmospheric carbon dioxide concentrations must therefore be accurate to one ppmv or even better.
Figure 1: Among U.S. greenhouse gas (GHG) emissions in 1990-2016, CO2 was the largest (81%).
In the past, CO2 concentration was often measured by lab analyses of grab-samples from the air near the Earth's surface. These measurements used mixtures of carbon dioxide and nitrogen for calibration standards, and accuracies of ± 0.2 ppm were achieved. This error at normal atmospheric CO2 concentrations was equivalent to ± 0.06% full-scale accuracy.
In addition to laboratory grab-sample analyses, continuous industrial CO2 measurements were made using portable or permanently installed non-dispersive infrared (NDIR) CO2 analyzers. The portable units are usually battery-powered, and their ambient sample is received by a combination of diffusion and convection effects in the sensor head, without any pumps or filtering. These units are provided with digital displays, one or two alarm settings, and analog or digital output signals. If gas filter correlation (GFC) is used in combination with single-beam, dual-wavelength designs, the analyzer is virtually immune to obstruction of the optics. This, in turn, prevents drift and thereby reduces the frequency of recalibration.
For very accurate atmospheric measurements, pulsing laser detectors (laser induced differential absorption radar, or LIDAR) are used. These operate at wavelengths slightly over 2 µm where CO2 is already highly absorbing, while H2O is not (Figure 2). The goal of wavelength selection is to minimize the overlap between the absorption bands of water vapor and CO2.
The use of grab-sample-type sensors is mostly limited to industrial applications. For monitoring carbon dioxide concentration in the atmosphere, the Total Carbon Column Observing Network (TCCON) was formed in 2004, and by 2015, consisted of 23 monitoring stations worldwide. TCCON is a global network of stations that measure the amounts of CO2, CO, methane, nitrous oxide and other trace gases in the atmosphere.
TCCON's goal is to investigate the flow (or flux) of carbon between the atmosphere, land and ocean (the so-called carbon budget or carbon cycle). This is achieved by measuring the atmospheric mass of carbon (the airborne fraction). TCCON measurements have improved the scientific community's understanding of the carbon cycle, and also the understanding of urban greenhouse gas emissions. TCCON can also assist the measurements that are made by airplane-, drone- or satellite-mounted systems. They do that by providing independent readings to compare with (or validate) space-based measurements when the satellite or airplane is flying over the TCCON site locations. For example, TCCON provides the primary measurement validation data set for the Orbiting Carbon Observatory (OCO-2) mission, and has been used to validate other space-based measurements of carbon dioxide.
NASA's role in CO2 measurements
In 2004, ground-based total column measurements mostly replaced the surface-only CO2 monitoring and later, those were supplemented by airplane, drone and, eventually, satellite monitoring. Space-based measurements of carbon dioxide help answer questions about Earth's carbon cycle. Remote sensing of trace gases has several challenges. Most techniques rely on observing IR light reflected off Earth's surface. Changes in surface albedo and viewing angles may affect measurements. Clouds may also interfere with accurate measurements, so platforms may include instruments to measure and compensate for clouds.
The first satellite mission was the Interferometric Monitor for Greenhouse Gases (IMG) onboard the ADEOS I satellite in 1996. This mission lasted less than a year. Since then, additional space-based measurements have been implemented, including those from two high-precision (better than 0.3% or 1 ppm accuracy) satellites (GOSAT and OCO-2).
Two decades of efforts by NASA in LIDAR and the "two-micron transmitter" development resulted in a new capability for remotely measuring the CO2 levels. NASA has developed integrated path differential absorption (IPDA) LIDAR, which incorporates high-energy, double-pulse lasers with high repetition rates. The laser pulses can be tuned and locked near the 2.05 micron wavelength, which is an ideal spectral region for CO2 sensing. Separated by 150 microseconds, the first pulse is tuned to a high CO2 absorption wavelength and the second pulse to a low absorption wavelength. By aiming the pulses at a hard target, the difference between the return signals correlates to the average amount of CO2 in the column between the instrument and the target.
NASA recently launched Orbiting Carbon Observatory-2 using passive monitoring, where CO2 measurements are made by observing the gas’ effect on sunlight. As sunlight passes through the atmosphere, CO2 and other molecules absorb light at distinct frequencies. The pattern of absorption can be as unique as a fingerprint. The intensity of the reflected sunlight from the Earth’s surface, which is partially absorbed by CO2 and oxygen, is recorded by the satellite and used to calculate how much carbon dioxide there is between the satellite and the surface. Measurements at different altitudes make it possible to monitor the global distribution of CO2 and its increase with the passage of time (Figure 2).
Figure 2: One infrared (IR) wavelength frequently used in CO2 measurements is slightly over 2 μm, where CO2 is highly absorbing and H2O is not.
The key limitation of passive measurements is that they can only operate in daylight, and it’s also necessary to gain a total, 24-hour understanding of the CO2 cycle. It’s important to know what’s happening at night because plants also "sleep" at night or in the winter. Their respiration also varies at high latitudes, including the poles, where in the winter there is no sunlight at all.
In contrast to passive monitoring systems that work with reflected sunlight, the ACES team is working with active monitors where the lasers send continuous-wave beams down to Earth. The ACES monitoring package is installed on an airplane that flies at about 30,000 feet. It has five lasers that can focus on the surface of the blue ocean below, using LIDAR to measure CO2. Unlike the passive Orbiting Carbon Observatory-2, ACES is "active" – its pulsed LIDAR beam concentrates all the laser energy into very short pulses of about one-millionth of a second each. This system generates the high laser power at precisely controlled frequencies that is needed for precise CO2 measurements of better than 1.0 ppmv (at today’s 410 ppmv, that is ±0.24%).
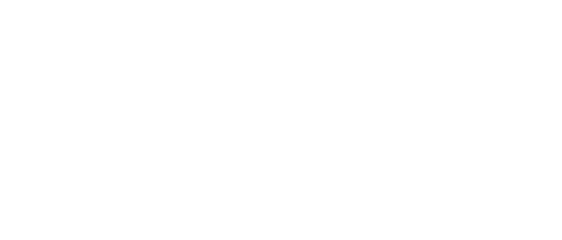
Leaders relevant to this article: