Air Liquide benefits from next-generation MPC
A PDF version of the full article is available for download here.
For more than 20 years, Air Liquide has leveraged multivariable model predictive control (MPC) technology to help optimize the performance of its air separation units (ASU). Many of these units are designed to rapidly adapt to real-time power pricing and changing customer demand. MPC helps, but the company realized its legacy MPC software was becoming increasingly costly to maintain and wasn't taking advantage of technological progress in the field.
Enter Yokogawa’s Platform for Advanced Control and Estimation (PACE), which was selected as the company’s new MPC standard. A multi-year campaign is now underway to deploy PACE at Air Liquide’s operational facilities in the U.S and worldwide. The platform’s unique and differentiating features solve many of the challenges that were inherent in Air Liquide’s legacy MPC software, and PACE is empowering Air Liquide’s control engineers to develop and deploy better control strategies for their increasingly autonomous ASUs.
Areas of notable performance improvement range from project execution workflow, plant efficiency and economics to sustainability of benefits and higher operations acceptance through minimal need for operator intervention during steady-state and rapid ramping of production rates.
A primer in air separation
Air Liquide is a world leader in delivering gas products, separation technologies and services to the chemical, energy, food, metals, medical, health and pharmaceutical industries. Oxygen, nitrogen and hydrogen are among the essential molecules for life, matter and energy, which in turn are embedded in Air Liquide’s scientific core and area of expertise since the company was founded in 1902.
An ASU (Figure 1) separates air into its main components—oxygen, nitrogen and argon—which are delivered to the company’s customers in gas and liquid states. The liquid products are held in storage tanks, then transported via truck. Gas products are supplied to customers via pipeline in local networks.
Electricity is the cryogenic ASU’s largest operating expense. The number of large compressors involved means that a single, large ASU can consume more than 50 megawatt-hours (MWh) of electricity.
Air Liquide adapts to customer demand and real-time energy pricing using several optimization strategies to make efficient and consistent production decisions. Overall production rate as well as product split is dictated by external factors such as customer demand and electricity price. Failure to quickly adapt to production demands will either result in venting products and unproductive power consumption, or necessitate the vaporization of stored product—liquid oxygen or liquid nitrogen from tanks.
A typical ASU is subject to a range of disturbances and demands that must be controlled, including changing production supply and demand, ambient weather conditions and front-end purification (FEP) cycle disturbances.
Ambient air temperature and relative humidity are typical disturbances to the ASU. Increasing air temperature will decrease inlet air density. Since centrifugal and axial compressor flow laws operate on actual volumetric flow through the compressor stage inlets, this will result in a reduced molar flow of air. Similarly, when relative humidity increases, the dew point rises and the cooling tower will produce hotter cooling/supply water, which in turn will result in higher interstage cooling temperatures, thereby reducing inlet density to later stages. Main air compressors feature inlet guide vanes to control these disturbances, but at high rates or warmer air temperatures, these will be saturated open and air throughput will be limited.
FEP-cycle disturbances are a type of periodic disturbance in a typical ASU. The FEP unit contains two dryer vessels that remove carbon dioxide and water from the air to prevent freezing in the cold-box. One dryer is online while the other dryer regenerates. Regeneration is done at high temperatures and low pressures, meaning the cycle affects the downstream process in a couple of ways. First, there's the introduction of a heat bump caused by the enthalpy of adsorption during repressurization when the regenerated dryer first comes online. This heat bump changes the thermal balance of the process, causing a disturbance. Second, and more significantly there's a loss of air, which needs to be replaced by the main air compressor and could cause a temporary decrease in product flows.
There's a wide range of dynamic response times in the ASU. Pressure effects are quick, thermal effects are slow, and the accumulation of argon in the distillation section is extremely slow. These varying transient dynamic responses, as well as tightly integrated distillation columns and heat exchangers, make it challenging to efficiently control an ASU. Traditional regulatory schemes for controlling product qualities require excessive operator interventions, especially during production rate ramping of the plant.
Next-generation MPC capabilities
PACE is a modern platform for advanced process control (APC) and estimation that was co-developed by Yokogawa and Shell, and released for commercial use in 2015. PACE enjoys a prestigious lineage. It's the successor to Shell Multivariable Optimization Controller (SMOC), which Shell used extensively in its refineries, hydrocarbon crackers and LNG plants.
SMOC was redesigned as PACE to overcome traditional APC challenges, to harness advancements in computing power, and to leverage learnings gained over 30 years of MPC design and operational experience. Today’s PACE platform achieves higher operational acceptance because it allows users to tailor and prioritize economics, as well as give operators better tools and insights. Improved performance is mainly due to a unique modeling structure that combines more than 10 advanced manufacturing software products into one platform. Further, it features two flexible workspace environments: off-line “design time” for design, testing and configuration of applications, and online “run time HMI” for deployment in the field.
Before the introduction of PACE, scripting was often required to achieve the custom functionality needed to control many processes. This scripting had to reside outside the MPC application, either in the DCS or another coding software added to the DCS logic. This approach introduced additional risk, took time and skill to implement, and created long-term maintenance issues as well. Air Liquide’s legacy MPC applications employed scripting to achieve many objectives, including automated step-testing; modification of models and tuning parameters based on plant mode; and custom control for specific scenarios like bed swapping, variable transformations and conditioning. With PACE this scripting is eliminated using standard product functionality.
The MPC modernization program at Air Liquide was undertaken in large part to better align operations with sustainability drivers, including:
- Avoid obsolescence of legacy software;
- Reduce required engineering support, while maximizing run time (i.e., eliminate need for excessive scripting);
- Provide integrated workflows with better tools to reduce the cost and time of deployment at new sites or retrofits;
- Use state-of-the-art algorithms to maximize process efficiency, particularly in large production units and multi-unit sites;
- Unify deployments on industry-standard software with global support, allowing different regions to share learnings, resources and reduce the overall cost of ownership; and
- Implement a versatile, multi-functional, integrated MPC platform that would complement and support Air Liquide’s remote operation strategy for continuously optimizing production efficiency and supply chain reliability with minimal operator intervention.
The results of Air Liquide’s evaluation and testing led them to select PACE as their next-generation MPC platform. A key differentiating characteristic of PACE is Shell's internal usage. This gave Air Liquide a level of comfort that development was being guided by operational feedback and would reduce the cost of ownership over the long term.
Model deployments
Air Liquide has been able to apply some of the new features of PACE to find new solutions to traditional MPC challenges. These reduced reliance on scripting, making the solutions more robust and sustainable.
One major differentiator is the ability of the control designer to specify the dynamic model structure to more accurately represent the actual system. Air Liquide’s legacy solution supported only a single layer of manipulated-variable (MV) and disturbance-variable (DV) inputs with dynamic finite-impulse-response (FIR) models identified as first-order-plus-deadtime. PACE, however, supports many different structural components. MV-to-CV (controlled variable) models can be composed with a variable number of layered intermediate variables (IVs). These IVs can be measured or calculated from a formula, and can be controlled (have CV relationships and constraints including high and low limits and setpoints) or uncontrolled. Additionally, models can either be dynamic, single-input-single-output (SISO), built out of 14 different types of transfer functions, or can be made of non-linear calculation blocks.
Base layer control (BLC) is a special subset of the IV concept. It's designed to model closed-loop relationships, such as the MV loop’s setpoint to the process variable (PV) and the setpoint to the output of a PID loop (OP). These relationships are present when MPC acts on top of PID loops in the DCS. PACE’s BLC handling adds additional benefits to a normal IV. BLC control handles the saturation of a valve or final control element. For example, in a flow control loop, it realizes that if the valve is saturated, increasing the flow setpoint will not result in additional flow (or any other process change for the matter). Instead, PACE will lower its setpoint to meet the measured flow even if other competing priorities call for additional flow.
This has the advantage that the MV setpoint “wind up” is prevented with respect to what the control valve can physically provide. Then, if it becomes necessary to decrease flow, the PACE can do so immediately without having to counteract many moves of windup. This feature significantly reduces the number of CVs traditionally added for managing the MV’s output valve physical limits. Additionally, BLC control properly handles disturbances of the PV away from the setpoint. Unlike with a normal IV, PACE knows that even if it takes no action, the PID controller (when not saturated) will move the loop output to bring the PV back to SP. Therefore, PACE adjusts its move plan accordingly and doesn't introduce a “double cascade correction” where both the outer (MPC) and inner (DCS) loop of a cascade scheme react and overcorrect for a disturbance on a MV loop.
In Air Liquide’s legacy MPC implementation, it was often useful to specify that certain MVs be used to control certain CVs. One reason is that some models are better (more consistently represent the process) than others. It's acceptable to use the imperfect MV-CV models as a feedforward disturbance, but better if the controlling MV has an accurate model. However, due to limitations in the previous generation of MPC technology, the way to make an MV act a disturbance to a CV was to add a DV that was identical to the MV, and add the relationship there (and delete it in original relationship), which caused a major disadvantage: the future trajectory of the MV was then not used to predict the future of CV. Only past moves of the MV were accounted for.
PACE provides the designer with the ability to select between “full control” or “prediction only” of the MV-to-CV relation for each SISO model element. An MV set as “prediction only” for a CV will be treated as a DV, and won't be included in the controller’s move plan for direct control of that CV, but it will be included in the estimation problem for the prediction of future trajectories. This results in a major benefit during production-rate ramping.
Traditional MPC solvers use a linear program to determine the best dynamic move plan of the MVs based on a fixed total number (N) of maximum discretized number of elements, or discrete points, to represent the near future. N is the maximum number of moves for each MV, and with Air Liquide’s legacy MPC it was limited to 10. Hence, the legacy MPC was short-sighted, leading to a negative impact, especially for slow CVs. This limitation of the number of future moves calculated by traditional solvers has many negative consequences, but two of the main ones are “jittery” control and controllers that fail to control the faster CVs in the presence of slower CVs in the same controller. For this reason, using the legacy MPC product required using two controllers on an ASU. These two sub-controllers had to interface with one another and required additional steps to setup properly, making it challenging and cumbersome.
PACE has no such limitation, featuring a plantwide estimation model (plant observer) that generates the open-loop prediction for the control model layer to solve a sequence of steady-state problems (where to go), followed by solving the dynamic optimization problem to determine the optimum move plan based on a full dynamic trajectory to steady state (how to get there) for each CV. The output results in a full set of optimum trajectories of the MVs, CVs and economic functions (EFs).
Figure 2: On a representative ASU, PACE APC implementation dramatically reduced the variability of process temperatures as well as stream flow rates and compositions.
More than promising results
Upgrading a representative ASU to the PACE platform showed argon yield improvement of 9%, along with a 30% reduction in standard deviation of the oxygen in the crude flow (Figure 2). The standard deviation on the argon yield CV improved by 42%, on top of an average increase of 9% in gaseous oxygen yields.
PACE’s Model Structure with Intermediate Variables (IVs) resulted in better model predictions, leading to better control performance and eliminating the need for operator interventions. This has led to higher overall acceptance of MPC operation. The improvement in the move plan’s smoothness and dynamic ability to adapt is clearly represented in Figure 2.
Air Liquide’s MPC sustainability project originally envisioned a two-phase execution approach:
- Phase I would be a feature-equivalent replacement of the legacy MPC at each facility to deal with challenges around impending obsolescence.
- Phase II would consist of going back to each facility that was migrated to PACE in Phase I, and enhancing the control performance using the new technology’s differentiating features.
Technically, Air Liquide is still in Phase I, but in practice, each replacement project has revealed new learnings and allowed improvements to the existing control schemes. PACE’s new features are already showing tangible economic benefits. Therefore, Phase II will consist of cycles of continuous improvement to MPC performance for higher operations acceptance. Also, though not expanded on here, PACE’s capabilities are proving applicable to—and paying dividends for—other Air Liquide technologies such as hydrogen production.
The promise of MPC is the ability to tell the MPC engine how the plant works and what the goals and constraints are, and let it worry about how to achieve them. Air Liquide’s legacy MPC software was never able to get to this level, mostly due to limitations with the model structure, solver and tuning that led the APC engineers to achieve their control objectives with time-consuming workarounds and extended maintenance support.
Figure 3: PACE's Model Structure with Intermediate Variables (right) resulted in better model predictions leading to better control performance and eliminating the need for operator interventions during even rapid production rate changes.
With PACE, MPC is very close to realizing this pure formulation for an ASU. Intermediate variables, non-linear blocks and BLC models make it possible to explicitly represent process knowledge. Constraints and non-linear economic objectives are easily expressed. With improved prediction-only control, an infinite horizon formulation and a nonlinear state-space solver, PACE provides smooth, adaptive, robust control—even with frequent changes in operating modes and production rates.
MPC is an established technology that's proven to provide significant benefits to the oil and gas, petrochemical and chemicals manufacturing industries. Yokogawa’s PACE improves MPC capabilities and usability from an operation and a deployment perspective, meaning the APC engineer can finally shift from working around software limitations to providing value to the organization. PACE is helping Air Liquide overcome the challenges posed by traditional MPC, allowing them to extend the boundaries of operation efficiency, reliability and profitability.
Download an expanded PDF edition of this article.
Bruce Eng is an international expert in smart manufacturing and process control at Air Liquide, with 13 years of experience in MPC, procedural automation and dynamic simulation.
Fady Kantara is APC principal consultant, KBC (a Yokogawa company) that leads the integration of PACE technology across customer plant sites.
Brian Burgio is APC program manager for KBC. He has over 30 years of international operational improvement experience in the chemical, petrochemical and refining industries.
Arnold "Marty" Martin, PE, CAP, is an international expert in smart manufacturing and process control at Air Liquide and director of process control technology.
Latest from Multivariable Control
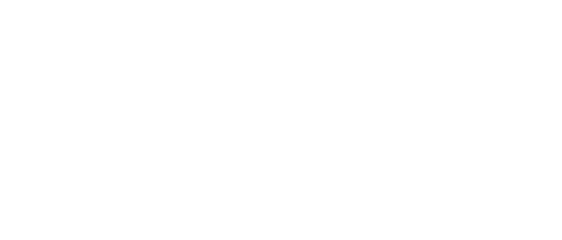
Leaders relevant to this article: